Occurrence, Fate, and Transport of Cryptosporidium parvum, E. coli
Occurrence, Fate, and Transport of Cryptosporidium parvum, E. coli O157:H7, Salmonella, Campylobacter, and indicator bacteria (Enterococcus spp, E. coli, Bacteroidales) in Porous Media (Groundwater,
Principal Investigators:
Thomas Harter (UC Davis), Rob Atwill (UC Davis), Aaron Packman (Northwestern University), Stephan Wuertz (UC Davis), Brian Bergamaschi (USGS)
Funding Sources:
USDA National Institute of Food and Agriculture,
California State Water Resources Control Board
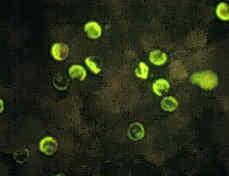
Furthermore, it has been suspected that recent food pathogen outbreaks (E. coli outbreak in spinach in 2006 and in salad in 2007, Salmonella outbreak in tomatoes, 2008) are potentially linked to irrigation water contamination with pathogens. This has greatly increased public interest and concern about the occurrence, fate, and transport of enteric bacteria and protozoa and their potential link to wildlife, animal farming, and human wastewater.
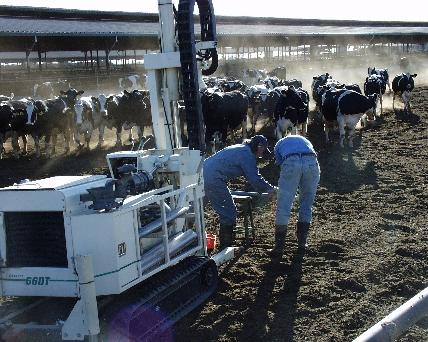
Animal husbandry has been a major suspect as a potential contamination source of enteric pathogens. To assess the public health risk from pathogens and their hydrologic pathways, we hypothesize that the animal farm is not a homogeneous diffuse source, but that pathogen loading to the soil and, therefore, to groundwater varies significantly between the various management units of a farm. A dairy farm, for example, may include an area with calf hutches, corrals for heifers of various ages, freestalls and exercise yards for milking cows, separate freestalls for dry cows, a hospital barn, a yard for collection of solid manure, a liquid manure storage lagoon, and fields receiving various amounts of liquid and solid manure. Pathogen shedding and, hence, therapeutic and preventive pharmaceutical treatments vary between these management units. We implemented a field reconnaissance program to determine the occurrence of three different pathogens (E. coli O157:H7 [groundwater only] , Salmonella, Campylobacter) and two indicator organism (Enterococcus, E. coli) at the ground-surface and in shallow groundwater of seven different management units on each of two farms, and in each of four seasons (spring/dry season, summer/irrigation season, fall/dry season, winter/rainy season). Results indicate that significant differences exist in the occurrence of these pathogens between management units and between organisms. These differences are weakly reflected in their occurrence in groundwater, despite the similarity of the shallow geologic environment across these sites. Our results indicate the importance of differentiating sources within a dairy farm and the importance of understanding subsurface transport processes for these pathogens. Further surveying of over 50 shallow monitoring wells in 8 dairy farms shows complete attenuation of E. coli O157:H7, very low frequency, sporadic detections of Salmonella, and possibly a seasonal breakthrough of Campylobacter into shallow, first-encountered groundwater. Domestic wells were pathogen-free, but had frequent detections of Enterococcus. Given the low frequency of pathogen detection, indicator organisms, in particular Enterococcus are possibly poor predictors of pathogen transport.
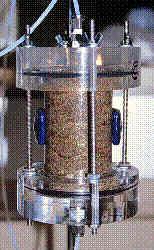
Table 1: Average relative velocity enhancement and mass retained in the soil column for each experiment. The relative total recovery is the sum % mass eluted (equal to the relative steady-state concentration at the bottom of the column) and the % mass retained. The sticking coefficient, , is computed after determining all other parameters independently. CS: coarse sand, MS: medium sand, FS: fine sand. ()* indicates questionable value due to experimental difficulties.
Experiment | CS fast | CS slow | MS fast | MS slow | FS fast |
velocity enhancement [%] | 16 | 27 | 8 | 10 | 0 |
mass retained [%] | 15 | 40 | 92 | 74 | 40 |
% total recovery | 84 | 50 | 93 | 74 | 41 |
sticking coefficient, | 4.8 | 2.6 | (6.6)* | 1.8 | 0.8 |
Outreach:
The principal investigators have given numerous presentations at stakeholder meetings, farm meetings, at workshops with consultants and animal farming industry personnel, and to local, state, and federal regulatory agencies. We have also presented much of our work at national and international scientific meetings. Part of our work is reflected in the U.S. EPA Long Term 2 Surface Water Treatment Rule of 2006, which provides criteria for Cryptosporidium parvum treatment credits given to bank filtration operations.
Publications:
Li, X., E.R. Atwill, E. Antaki, O. Applegate, B. Bergamaschi, R.F. Bond, J. Chase, K.M. Ransom, W. Samuels, N. Watanabe, and T. Harter, 2015. Fecal indicator and pathogenic bacteria and their antibiotic resistance in alluvial groundwater of an irrigated agricultural region with dairies. J. Env. Qual. 44:1435-1447, doi: 10.2134/jeq2015.03.0139 (open access).
Bradford, S.A., J. Schijven, and T. Harter, 2015. Microbial transport and fate in the subsurface environment: Introduction to the special section. J. Env. Qual. 44:1333-1337, doi: 10.2134/jeq2015.07.0375.
Harter, T., N. Watanabe, X. Li, E. R. Atwill, and W. Samuels, 2014. Microbial groundwater sampling protocol for fecal-rich environments, Groundwater, doi:10.1111/gwat12222 (open access).
Li, X., N. Watanabe, C. Xiao, T. Harter, B. McCowan, Y. Liu, E. R. Atwill, 2013. Antibiotic-resistant E. coli in surface water and groundwater in dairy operations in Northern California. Environ. Monit. Assess, doi:10.1007/s10661-013-3454-2 (pdf file for personal use only).
Park, Y., E.R. Atwill, L.L. Hou, A.I. Packman, and T. Harter, 2012. Deposition of Cryptosporidium parvumoocysts in porous media: A synthesis of attachment efficiencies measured under varying environmental conditions. Env. Sci. Tech. 46 (17), pp. 9491–9500, doi:10.1021/es300564w (free public access).
Bremer, J. and T. Harter, 2012. Domestic wells have high probability of pumping septic tank leachate, Hydrol. Earth Sys. Sci 16:2453-2467, doi:10.5194/hess-16-2453-2012 (free public access).
Unc, A., M. J. Goss, S. Cook, X. Li, E. R. Atwill, and T. Harter, 2012. Analysis of matrix effects critical to microbial transport in organic waste-affected soils across laboratory and field scales. Water Resour. Res. 48, W00L12, 17p., doi:10.1029/2011WR010775.
Harter, T., E.R. Atwill, L.L. Hou, B.M. Karle, and K.W. Tate, 2008. Developing risk models of Cryptosporidium transport in soils from vegetated, tilted soilbox experiments. J. Env. Qual. 37(1), 245-258. doi:10.2134/jeq2006.0281.(pdf file for personal use only)
Cortis, A., T. Harter, L. L. Hou, E. R. Atwill, A. I. Packman, P. G. Green, 2007. Transport of Cryptosporidium parvum in porous media: Long-term elution experiments and continuous time random walk filtration modeling. Water Resour. Res. 42(12), W12S13, doi:10.1029/2006WR004897.
Searcy, K.E., A. I. Packman, E. R. Atwill, and T. Harter, 2006. Deposition of Cryptosporidium oocysts in streambeds. Applied and Environmental Microbiology, 72(3):1810-1816. (pdf file for personal use only)
Discussion of C. parvum removal in bank filtration
Searcy, K. E., A. Packman, E. R. Atwill, and T. Harter, 2005. Association of Cryptosporidium parvum with Suspended Particles: Impact on Oocyst Sedimentation, Applied and Environmental Microbiology 71(2):1072-1078.(pdf file for personal use only).
Atwill, E. R., L. Hou, B. M. Karle, T. Harter, K. W. Tate, R. A. Dahlgren. Transport of Cryptosporidium parvum oocysts through vegetated buffer strips and estimated filtration efficiency, Applied and Environmental Microbiology 68(11), pp. 5517-5527, 2002.(pdf file for personal use only).
Harter, T., S. Wagner, E. R. Atwill, Colloid transport and filtration of Cryptosporidium parvum in sandy soils and aquifer sediments, Env. Science and Technology, 34(1), 62-70, 2000. (pdf file and supplement for personal use only).